Computational Condensed Matter and Nanoelectronics
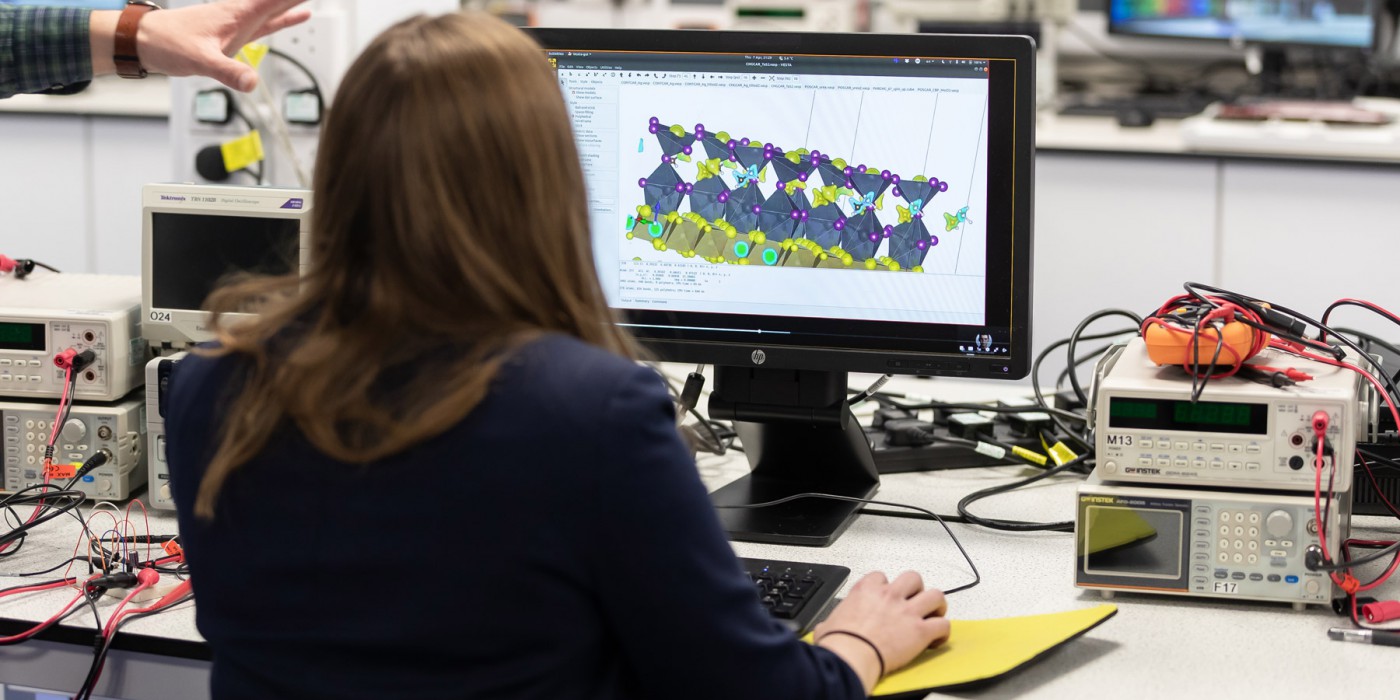
The CCMN research performs state-of-the-art ab initio computational modelling that discerns the underlying quantum mechanical nature of organic and crystalline materials as well as heterostructures, from the point of view of fundamental physics and technological applications in nanoelectronics and optoelectronics.
Research
At CCMN, our research efforts focus on the following scientific areas that span across condensed matter physics, solid state physics, materials science and nanoelectronics. We are always looking for new research collaborations, so please get in touch if you are interested in working with us.
Electronic structure of materials surfaces and interfaces
In electronic and optoelectronic devices, such as field-effect transistors, solar cells and light emitting diodes, it is crucial to optimise the energy level alignment between materials interfaces, in order to improve device efficiency. We work in close contact with experimental groups that are experts in device fabrication and use our computational methodology complementary to acquire deeper understanding of the interplay between materials interface science and device physics in the presence of defects and impurities. We use a combination of density functional theory and Poisson equation to quantify charge transfer between thin film layers in the device. Such layers can be either conducting or semiconducting. Upon the creation of the interface, we investigate i) the appearance of gap states in the n- or p-type semiconductor, ii) the occurrence of Fermi-level pinning, iii) the work function modification of the metal substrate due to the formation of the metal/semiconductor interface, and iv) the consequent effect on energy level alignment at the very interface. Agreement is sought between theory and experiment via UPS and XPS measurements. Our computational methodology provides insight into how the work function of the anode and cathode could be tuned to provide efficient electron injection/collection and finally be transformed into highâperformance optoelectronic device electrodes. Selected publications in this area are as follows:
- Y. Zhou et al. Science 336, 327 (2012).
- T. A. Papadopoulos et al. Adv. Funct. Mater. 23, 6091 (2013).
- E. Polydorou et al. Nano Energy 34, 500 (2017).
- M.-K. Lin, et al. Phys. Rev. B 95, 085425 (2017).
Please contact Dr Theodoros Papadopoulos for further information.
Charge and exciton transport in organic thin films
Our work is related to applications such as Organic Photovoltaics (OPVs), Organic Light Emitting Diodes (OLEDs), and Organic Field-Effect Transistors (OFETs). The theoretical methodology we use is based on the combination of Molecular Dynamics to predict the morphology of organic thin films and Kinetic Monte Carlo modelling, parametrised via ab initio methods, to simulate electron, hole, or exciton transport. We carry out a systematic comparison with available experimental data, and analyse the role that the dynamic nature of positional and energetic disorder play on the temperature and electric field dependence of charge mobility, or exciton diffusion length. Our work establishes a clear link between microscopic and macroscopic transport quantities and utilises nanoscale theoretical schemes as a tool for predictive material screening towards efficient organic optoelectronic applications. Selected publications in this area are as follows:
- T. A. Papadopoulos et al. Chem. Sci. 2, 1025 (2011).
- S. M. Gali et al. J. Chem. Phys. 147, 134904 (2017).
Please contact Dr Theodoros Papadopoulos for further information.
Molecular electronics
Using a first-principles approach that combine density functional theory, scattering theory and Green functions within the Landauer-Buttiker formalism, we study the electron transport properties of molecular wires, which may form the interconnects for future molecular electronics devices. Conductance and IV curves are extracted to characterise a wide range of molecular nanodevices. Via our methodology, the occurrence of Breit-Wigner and Fano resonances are explored to identify whether electron transport can be controlled via imposing conformational modifications on the molecular wire itself, acting hence as a molecular diode or sensor. Selected publications in this area are as follows:
- T. A. Papadopoulos et al. Phys. Rev. B 74, 193306 (2006).
Please contact Dr Theodoros Papadopoulos for further information.
Quantum Monte Carlo Modelling
We are also engaged in fundamental computational research exploring the electron-electron interaction, in the homogeneous electron gas (HEG), employing the quantum Monte Carlo technique. The HEG consists of a sea of electrons together with a uniform positive background charge density. Information gleaned from numerical studies of the HEG form the basis of the exchange-correlation functionals, used in almost all density functional theory simulations of real materials. We also investigate the Coulomb attraction between electrons and holes in layered semiconductor systems. Our calculations show that at low electron densities isolated few-body species are present, while at higher electron densities collective, many-body behaviour is observed. Selected publications in this area are as follows:
- G. G. Spink et al. Phys. Rev. B 88, 085121 (2013).
- G. G. Spink et al. Phys. Rev. B 94, 041410(R) (2016).
Please contact Dr Graham Spink for further information.
Quantum Dot Cellular Automata
QCA devices and circuits are a brand-new paradigm for computer architecture. QCA are transistorless and the charge configuration of quantum dots encodes binary information. In particular, we perform numerical calculations for the switching of QCA mixed valence molecule and simulate the Clocking of Molecular QCA, by lithographically defined wires, buried in the substrate, with the molecules located at the surface. Selected publications in this area are as follows:
- M. Alharbi et al. Nanomaterials 13, 2445 (2023)
- M. Alharbi et al. J. Supercomput. 79, 11530 (2023)
Please contact Dr Richard Stoker and Dr Gerard Edwards for further information.
Students
MRes and PhD students who would like to work with us are advised to first identify a CCMN area of research interesting to them and then contact our research academics to discuss the possibility of formulating a research project of their interest. Submitting research proposals with motivated students is always within our interest, so please do get in touch!
PhD and MRes students in the CCMN group are as follows.
Exton Park is centrally located in Chester, and accessible via the M53, A483, A41, A56, and public transport from the city centre.
Find your way around Exton Park
Address
University of Chester, Alexandra House, CAX202, Parkgate Road, Chester, CH1 4BJ
Cycling
The Greenway Gate, accessible between 7am – 9pm with your University pass, is located at the back of the overflow car park and is the nearest point of access to the Millennium Greenway cycle path that connects to routes throughout the city.
Public Transport
Bache train station is a 15-minute walk from Exton Park and Chester railway station is a 20-minute walk. Arriva 1A and Arriva 1 run frequent services that stop with a short walk to Exton Park. All three Park and Ride routes, PR1, PR2 and PR3 have connections within walking distance to Exton Park.
Parking
Parking on campus is limited, so we recommend using public transport and/or walking if possible.